Investigating the Multidrug Resistance of MRSA and the Genes Involved
- 이수정
- 2015년 8월 18일
- 14분 분량
이수정
Abstract: MRSA, which stands for Methicillin Resistant Staphylococcus Aureus, is a strain of the bacterium Staphylococcus Aureus which is a coagulase positive gram positive bacterium. It is very dangerous, resistant to drugs, and can even cause serious, life-threatening problems. This study is focused on identifying the gene that is related to drug resistance. For the investigation of the multidrug resistance of MRSA, a sample of MRSA was obtained from a local hospital. The DNA from MRSA was extracted and mixed with E. coli for transformation of the host bacteria. The resistance of the transformed E. coli was tested in different concentrations of ampicillin, gentamycin, kanamycin, and tetracycline. To investigate the genes that were involved in the multidrug resistance, I first prepared the transformed E. coli via mini prep, and then ran the E. coli through PCR. Finally, the method of gel electrophoresis was used to identify the genes involved in drug resistance. According to the results, both MRSA and the genetically transformed E. coli show drug resistance to some antibiotics. It can be interpreted that the DNA that codes for antibiotic resistance was successfully transported into the E. coli that survived the antibiotics. The DNA was amplified in both the drug resistant MRSA and E. coli through PCR. Since the section of DNA that codes for the multidrug resistance is most likely shared in all the bacterium that survived the antibiotics, the DNA involved in drug resistance can be identified through electrophoresis.
Keywords: MRSA (Methicillin Resistant Staphylococcus Aureus), Multidrug resistance, ampicillin, gentamycin, kanamycin, tetracycline
I. Introduction
1. The Issue of MRSA
Issue ofMRSA and non-MRSA (or MSSA: Methicillin sensitive S. aureus) are differentiated by the resistance to methicillin, but methicillin resistance is not the most important aspect about MRSA. What really makes MRSA stand out from other S. aureus is that it exhibits resistance to β-lactam antibiotics. Methicillin is a β-lactam antibiotic, and is used commonly for finding MRSA. Because of its multidrug resistance due to evolution, MRSA is a serious problem in the medical community.
MSSA, the strain of staphylococcus that is sensitive to β-lactam antibiotics, was not considered a serious threat, but in the last 50 years, mutations led to MRSA, which was much more dangerous than the original staphylococcus aureus. Originally, staphylococcus aureus was not contagious, but MRSA is very contagious with high frequencies of nosocomial infections.
2. Purpose of Research
The experiments were performed under three purposes. First, to investigate the multidrug resistance of MRSA and roughly understand the DNA involved in the multidrug resistance. Second, to investigate the validity of the currently used methods. Finally, to pave way for the development of a MRSA-sensitive drug in the long run.
3. Research Methods and Data Gathering
To investigate the multidrug resistance of MRSA, a sample of MRSA was obtained from a local hospital. Then, the DNA from the MRSA was extracted and mixed with the prepared E. coli, which would hopefully cause transformation. Now, the E. coli should show the same resistance to drugs that the DNA from the original MRSA coded for. Thus, the resistance of MRSA could be investigated by attempting to grow the transformed E. coli in a culture of different antibiotics in different concentrations, and observing its growth. The resistance of the transformed E. coli was tested in different concentrations of ampicillin, gentamycin, kanamycin, and tetracycline. To identify the genes involved in the multidrug resistance, the transformed E. coli was first prepared via mini prep, followed by running the E. coli through PCR. This should multiply the number of DNA in the E. coli, allowing investigation of the genes. The final step was running the process of gel electrophoresis. The theoretical background knowledge was gathered from perusing various articles.

Fig. 1 Diagrams of how PCR works (left) and gel electrophoresis (right)
II. Theoretical Background of Research
I. The Antimicrobial Resistance of Staphylococcus Aureus
In the early 1970s, physicians were finally forced to abandon their belief that, given the vast array of effective antimicrobial agents, virtually all bacterial infections were treatable.1 Their optimism was shaken by the emergence of resistance to multiple antibiotics among such pathogens as Staphylococcus aureus, Streptococcus pneumoniae, Pseudomonas aeruginosa, and Mycobacterium tuberculosis. The evolution of increasingly antimicrobial resistant bacterial species stems from a multitude of factors that includes the widespread and sometimes inappropriate use of antimicrobials, the extensive use of these agents as growth enhancers in animal feed, and with the increase in regional and international travel, the relative ease with which antimicrobial-resistant bacteria cross geographic barriers.2,3,4
Nowhere has this issue been of greater concern than with the Gram-positive bacteria pneumococci, enterococci, and staphylococci. Multidrug resistance is now the norm among these pathogens. S. aureus is perhaps the pathogen of greatest concern because of its intrinsic virulence, its ability to cause a diverse array of life-threatening infections, and its capacity to adapt to different environmental conditions. The mortality of S. aureus bacteremia remains approximately 20–40% despite the availability of effective antimicrobials. S. aureus is now the leading overall cause of nosocomial infections and, as more patients are treated outside the hospital setting, is an increasing concern in the community.5,6,7
S. aureus isolates from intensive care units across the country and from blood culture isolates worldwide are increasingly resistant to a greater number of antimicrobial agents. Inevitably this has left fewer effective bactericidal antibiotics to treat these often life threatening infections (Figure 1). As rapidly as new antibiotics are introduced, staphylococci have developed efficient mechanisms to neutralize them (Table 1). Recent reports of S. aureus isolates with intermediate or complete resistance to vancomycin portend a chemotherapeutic era in which effective bactericidal antibiotics against this organism may no longer be readily available. This review will focus on the emergence of antimicrobial resistance in S. aureus. It will review the historical evolution of resistant strains, their spread, the molecular mechanisms of resistance for selected antibiotics, and progress toward the development of alternative drug targets or novel approaches for therapeutic or prophylactic intervention.8,9,10,11
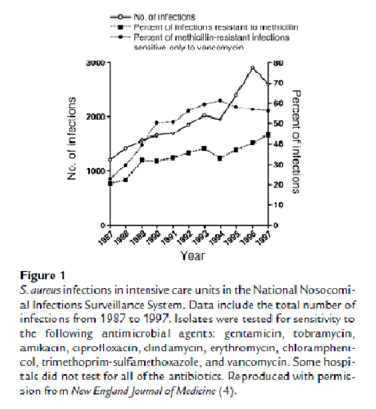
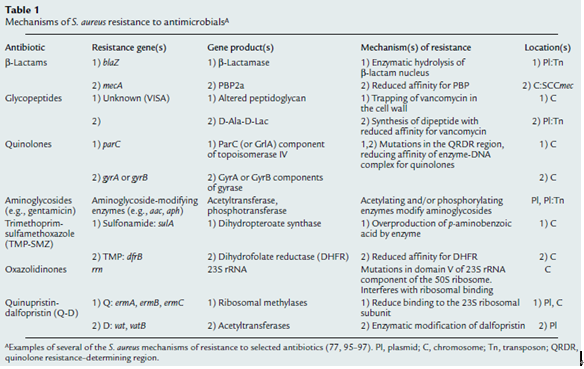
III. Experimental Methods
1. Extracting the MRSA gene
The gene from the MRSA sample that had been obtained from a hospital had been preserved in a petri dish in advance. The petri dish containing the frozen MRSA was opened very carefully, and then I scraped some of the MRSA with a disposable inoculating loop. Ater placing the MRSA in LB liquid broth, the liquid broth with the MRSA was placed in a shaking incubator for 24 hours.
In order to observe the resistance of MRSA to antibiotics, as the next step I dropped small amounts of MRSA in LB solid plates that each had an antibiotic in it. These were again situated in an incubator for 24 hours. After the completion of the whole process, I was able to observe the subsequent growths

Fig3. Samples of antibiotics, placing the MRSA in liquid broth
2. Transformation
Transformation happens when a cell's genetic material is altered through uptake of foreign DNA. Sometimes, transformation happens naturally when bacteria are transformed without much outer interference. By utilizing the method of transformation, I attempted to transform E. coli with no drug resistance with the DNA of the MRSA extracted by miniprep. 50uL of the competent cell* which had been kept in -80°C was first taken out and melt in ice. 1uL of plasmid DNA** was also added to each tube, mixed well and placed in ice for 30 minutes.

Fig4. competent cell and plasmid DNA (left), adding plasmid DNA (right)
Afterwards, the plasmid DNA was placed in the competent cell and kept in 42°C for 30 seconds and then relocated in ice for 2 minutes. 250uL of LB liquid broth was then added to these in different falcon tubes, and then put in a shaking incubator for an hour under 37°C. Only 100uL was left, and the rest was disposed.
After resuspending the cell, 25uL of the remaining solution was spread on each of the ampicillin, kanamycin, gentamycin, and tetracycline LB solid plates. 25uL of competent E. coli was spread on the LB solid plates with the same antibiotics.
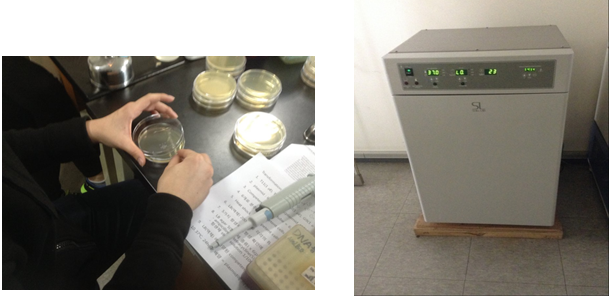
Fig5. spreading competent E. coli (left), incubator (right)
I waited until the E. coli bacteria smeared into the plate, and once again, grew the resulting cells in an incubator for a day under 37°C. Finally, the miniprep process was repeated.
3. PCR
Polymerase Chain Reaction, or PCA is a technique used to amplify a certain section of DNA. I used PCR in order to amplify the DNA I had obtained from the transformed E. coli in order to analyze the DNA. Because it is much easier to analyze DNA in large quantities, it PCR is known to be frequently used before DNA analysis.
As the first step to using the PCR machine, I added 10uL of SYBR Green I, a cyanine dye used as a nucleic acid stain, to each PCR tube. I then added 0.3uL of each primer, which is necessary for DNA replication, to each PCR tube.
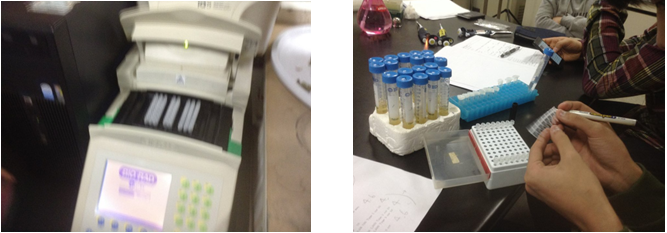
Fig6. PCR machine (left), labeing the PCR tubes (right)
Then, in order to dissolve the dry primers, the process of dilution with distilled water had to be undergone until the mixture reached 19.5uL. After 0.5uL of the sample DNA was added to each PCR, the PCR machine was run.
4. Gel Electrophoresis
As the last method, I used gel electrophoresis to analyze the DNA that was extracted from the transformed E. coli and amplified through PCR. By the following steps, I analyzed several DNA strands that had been attained from the cells that showed multidrug resistance.
I first put 1g of Nusieve® GTG® Agarose in an Erlenmeyer flask. In the same flask, I put 1g of Seakem® LE Agarose. Then, I added 1xTBE until the 100ml mark on the Erlenmeyer flask was reached. The flask was heated in a microwave oven for 2 minutes
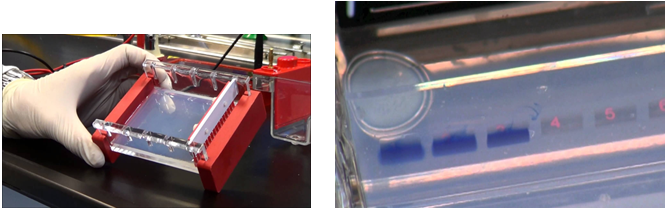
Fig7. gel electrophoresis mold (left) wells with markers loaded in them (right)
After the gel was cooled, I slowly poured it into the gel electrophoresis mold. I added a comb to the mold on the side, so that the gel would form walls. In the first well, I loaded 3uL of the marker* ; I mixed 3uL of each sample with stain** and loaded them into other wells. After loading into all the wells, I, connected the mold to a circuit, confirming that the mixture moved down to the bottom. The results could be observed by putting in a Gel image analyzer.
IV. Results
The MRSA was first grown in liquid LB broth, and then placed in solid LB plate with a variety of antibiotics. The following results of the growth of the MRSA were observed.
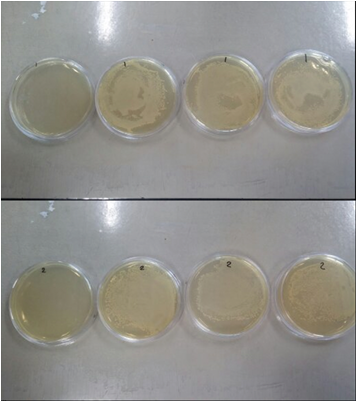
Figs 8. The petri dishes on the top are the resulting growth of MRSA from patient 7, and the dishes on the bottom are the resulting growth of MRSA from patient 8. The antibiotics used are tetracycline, kanamycin, gentamycin, and ampicilin, left to right.
The results show that MRSA has resistance to gentamycin, ampicillin, and kanaycin, but slight or no resistance to tetracycline.
Then, I wanted to transform the E. coli with the DNA from the MRSA and test its resistance, but first, I had to test the original E. coli in the drugs, as without this, it would be impossible to know whether the drug resistance is from the MRSA DNA or from the E. coli. The E. coli was placed on a LB plate with several antibiotics and its growth was observed; this being the control of this section of the experiment. The photograph below shows the resulting growth of the E. coli- the E. coli has no resistance to gentamycin, kanamycin, ampicillin and tetracycline.
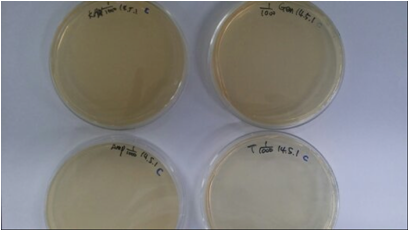
Fig9. Growth of E. coli 24 hours after it was placed in different antibiotics
(top left: kanamycin, top right: gentamycin, bottom left: ampicillin, bottom right: tetracycline) with 1/1000 concentrations
Then, to test the drug resistance of the transformed E. coli, I placed the E. coli in a LB plate and observed its growth. The resulting growth of the transformed E. coli is as shown below.
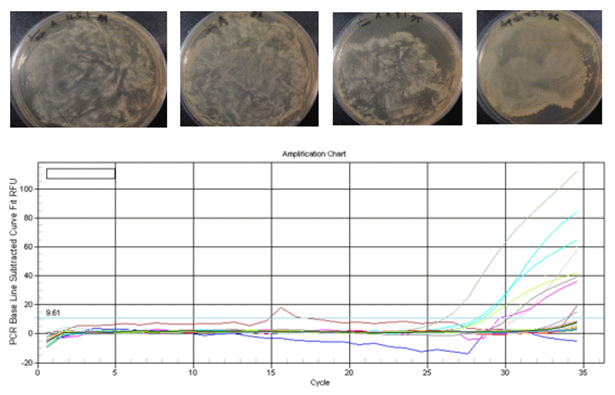
Figs 10.
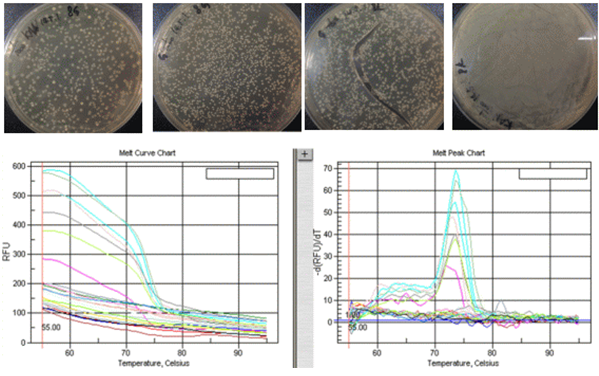
Figs 11.
The graphs above are the graphs that resulted after performing PCR with a variety of primers and DNA. The PCR machine can measure the progress of PCR by measuring the fluorescence; the following are the PCR program and lane placements.
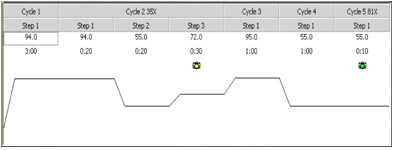
Fig12. PCR program
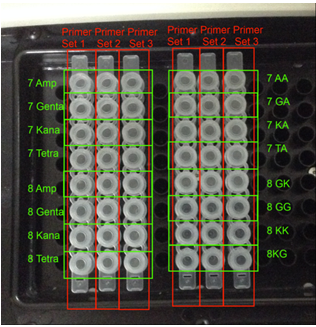
Fig13. PCR lane placements in my experiment
The graph in Figs10 is the amplification chart of the DNA. The x-axis is the number of cycles the DNA went through, and the y-axis is the amount of DNA measured by the fluorescence level. A successful PCR starts to grow exponentially at about 20-25 cycles, while flat lining curves represent DNA that is not copied very much.
The graphs in Figs11 are the melt curves and the melt peaks. The x-axis is the temperature, and the y-axis is is the flurorescence level which represents the number of associated double strand DNA (as opposed to dissociated single strand DNA). By taking the first derivate of the melt curve, we can get the melt peak chart. Melt curves with steep slopes mean there are more DNA there to be dissociated, which means that the PCR was successfully done. since the melt peaks are the first derivation of melt curves, high melt peaks mean steep slopes, and therefure successful PCR. I decided to conduct gel electrophoresis on the DNA with the highest melt peaks.
I then analyzed the amplified DNA using gel electrophoresis. The primers used in PCR copies the DNA at different sites, so if the bands from DNA amplified using the same primer results in similar bands, that most likely means that the section of the DNA is included in both of the stands of DNA. This leads to the interpretation that the section of DNA that is shared in the DNA segments with multidrug resistance could code for multidrug resistance.

Fig14.
7 A, G, K, T: DNA of MRSA from patient 7 grown in ampicillin, gentamycin, kanamycin and tetracycline, respectively.
8 A, G, K: DNA of MRSA from patient 8 grown in ampicillin, gentamycin, kanamycin and tetracycline, respectively.
7GA, KA, TA: DNA of E. coli grown in ampicillin, which were transformed with the DNA of MRSA from patient 7 grown in gentamcycin, kanamycin, and tetracycline, respectively.
8 GK, KK: DNA of E. coli grown in kanamycin, which were transformed with the DNA of MRSA from patient 8 grown in gentamycin and kanamycin, respectively.
8 GG: DNA of E. coli grown in gentamycin, which were transformed with the DNA of MRSA from patient 8 grown in gentamycin.
The investigation showed that MRSA showed resistance to some of the antibiotics, and some of the transformed E. coli showed antibiotic resistance. That most likely means that the DNA that codes for antibiotic resistance was successfully transported into the E. coli that survived the antibiotics. By running PCR, some of the DNA in the drug resistant MRSA and transformed E. coli could be amplified. Since the section of DNA that codes for the multidrug resistance is most likely shared in all the bacteriumthat survived the antibiotics, by analyzing the DNA through gel electrophoresis, it was possible to get an idea about which section of DNA was shared.
V. Discussion
1. MRSA - What options does a clinician have?
There are options for the treatment of MRSA but they are not without limitations. One can arbitrarily divide the options into short-term, mid-term, and long-term options.
The immediate or short-term options include:
•More diligent and improved infection control as the spread of MRSA is linked directly to suboptimal procedures in the hospital setting where MRSA can spread clonally by lack of hand-washing, inadequate disinfection, breakdown insterile field containments etc.
•To accomplish #1, improvements in the rapid diagnosis of MRSA must be made towards 100% accuracy and detection levels;
•More judicious usage of existing anti-staphylococcal antibiotics in both the ICU and non-ICU setting.
For mid-term efforts, the hope is:
•A more definitive understanding of MRSA reservoirs in the hospital setting, and the implementation of appropriate infection control measures.
•Completion of development and approval/launch of the late-stage compounds in clinical development that target MRSA infections.
•Improved understanding of the potential molecular mechanisms of MRSA resistance and strategies to reduce the risk of resistance with existing knowledge (e.g., combination or synergism therapy, PK/PD considerations in dosing for the ‘ure’ mutant prevention concentration etc.)
For the longer-term efforts, there are several key parts:
•Increased drug discovery efforts towards the discovery of new anti-MRSA agents.
•Development of specific anti-MRSA drugs and staphylococcal vaccines, as well as novel genomic target-based chemical classes to combat MRSA resistance. This may be either by targeting an MRSA-specific essential gene or the identification of adjunct therapy (non-antibiotics) that restore drug susceptibility to MRSA or reduce
pathogenicity.
2. Expert Opinion
The appearance of MRSA in the clinic almost 45 years ago began a slow, progressive selection of resistance in S. aureus that has brought us to this point of MRSA being a major nosocomial pathogen and a new problem emerging with CA-MRSA. One can most likely expect the resistance problem to intensify within the clinic over the next 5 –10 years. The evolution of MRSA resistance, measured by both microbiological susceptibility and molecular analyses of global clones indicates multiple, independent occurrences of the SSCmec genetic element, presumed to be acquired from another Gram-positive micro-organism. As this once ‘minor’ pathogen, in its fully susceptible phenotype, became resistant to one drug after the other, its relevance in the clinic has increased to today’s point of being a major concern to ID specialists. The related S. epidermidis pathogen may follow the same evolutionary pathway of MRSA, which will only confound treatment. Although there are limited options for empirical therapy of MRSA, the arsenal for treatment of CA-MRSA has more weapons; but the susceptibility pattern is no longer guaranteed, and the trend towards loss of susceptibility is not encouraging. Fortunately, there is growing recognition of this existing HA- and emerging CA-associated problems of MRSA, and the handful of hospital agents will soon be supplemented with several new anti-MRSA drugs. In addition, there are other ongoing efforts to intervene, both prophylactically (i.e., vaccine) and postinfection (new class anti-MRSA agents) on the horizon. But what will we do if CA-MRSA continues to increase and becomes an MDR CA-MRSA?
Beyond these imminent advancements, the extensive knowledge of the microbiological susceptibilities of MRSA, biochemistry and physiology, and molecular biology and genetics has provided multiple other options for approaching this problem. Taken together en mass, and with multiple scientific confirmations of the need and importance of establishing and maintaining good infection control in the hospital, the prospects of control of MRSA in the clinic may be more optimistic than previously thought. However, once MRSA is under control, history predicts that another opportunistic pathogen will emerge, and the process will begin again with the emergence of the next potential ‘superbug’.
3. Further Outlook
Based on the data gathered so far, the next possible step would be to analyze the sequence of the section of DNA, but I was not able to precede that far. Other than the DNA sequence coding, another experiment to execute based on this study would be to use Quorum Sensing to detect the genes that contribute to drug resistance within MRSA.
Quorum sensing is a method of bacterial communication that is performed through an inducer and a receptor. All bacteria are capable of Quorum Sensing, and the significance of using this technique is that not only does it make the antibiotic resistant genes more easily detectable, but it also makes the process faster. The hypothesis is that if a bacteria number 2 is used with the reporter gene GFP/ lacZ/ luciferase, it would be possible to recognize the existence of antibiotic genes in the bacteria number 1 depending on what bacterial antibiotics are put in. This is because as the antibiotics root out the bacteria number ones without resistance, only the genes of the resistant ones are expressed and reported by the second bacteria.

Fig15. An example diagram of Quorum Sensing (Tinkercell)
For Quorum Sensing, AHL, plasmids, and two bacterial strains would be needed- one for the reporter and the other for the tester bacteria. First, some gene transfers would have to be performed to tailor the bacteria to catered needs, such as the reporter bacteria having the needed GFP plasmid. Then, it can be moved on to exposing the tester bacteria to various antibiotics to test whether the antibiotic resistance gene is there or not. There still are some anticipated problems such as whether this system is really an accurate measurement of antibiotic resistance in the family of bacteria with antibiotic resistance and whether there would be a proper method of measuring the reporter gene. However, since successful utilization of Quorum Sensing will help pave way for further antibiotic development, this method for detecting the antibiotic resistant genes of MRSA is worthy of research.
VI. References
1. CHOPRA I: Antibiotic resistance in Staphylococcus aureus: concerns, causes and cures. Expert Rev. Anti-infect. Ther. (2003) 1(1):45-55.
2. SOLOMON S, HORAN T, ANDRUS M et al.: National Nosocomial Infections Surveillance (NNIS) System Report, data summary from January 1992 through June 2003, issued 2003. Am. J. Infect. Control (2003)31:481-498.
3. ANONYMOUS: Proportion of S. aureus nosocomial infections resistant to oxacillin (MRSA) among intensive care unit patients, 1989-2003. NNIS System, 2004.
4. BARRETT CT, BARRETT JF: Antibacterials: are the new entries enough to deal with the emerging resistance problems? Curr. Opin. Biotechnol. (2003) 14:1-6.
5. WALSH C: Where will new antibiotics come from? Nat. Rev. Microbiol. (2003) 1:65-70.
6. LIVERMORE DM: The threat from the pink corner. Annals Med. (2003) 35:226-234
7. BUSH K, MACIELAG M, WEIDNER-WELLS M: Taking inventory: antibacterial agents currently at or beyond Phase I. Curr. Opin. Microbiol. (2004) 7:466-476.
8. BUSH K: Why it is important to continue antibacterial drug discovery. ASM News (2004) 70(6):282-287.
9. PROJAN S: New (And Not So new) Antibacterial Targets –From where and when will the novel drugs come? Curr. Opin. Pharmacol. (2002) 2:513-522.
10. SHLAES D: The abandonment of antibacterials: why and wherefore? Curr. Opin. Pharmacol. (2003) 3(5):470-473.
11. SHEAGREN JN: Staphylococcus aureus: the persistent pathogen (part 1). New Engl. J. Med. (1984) 310:1368-1373.
Comments